AN INTUITIVE LOOK AT ELECTROCHEMICAL IMPEDANCE SPECTROSCOPY (EIS)
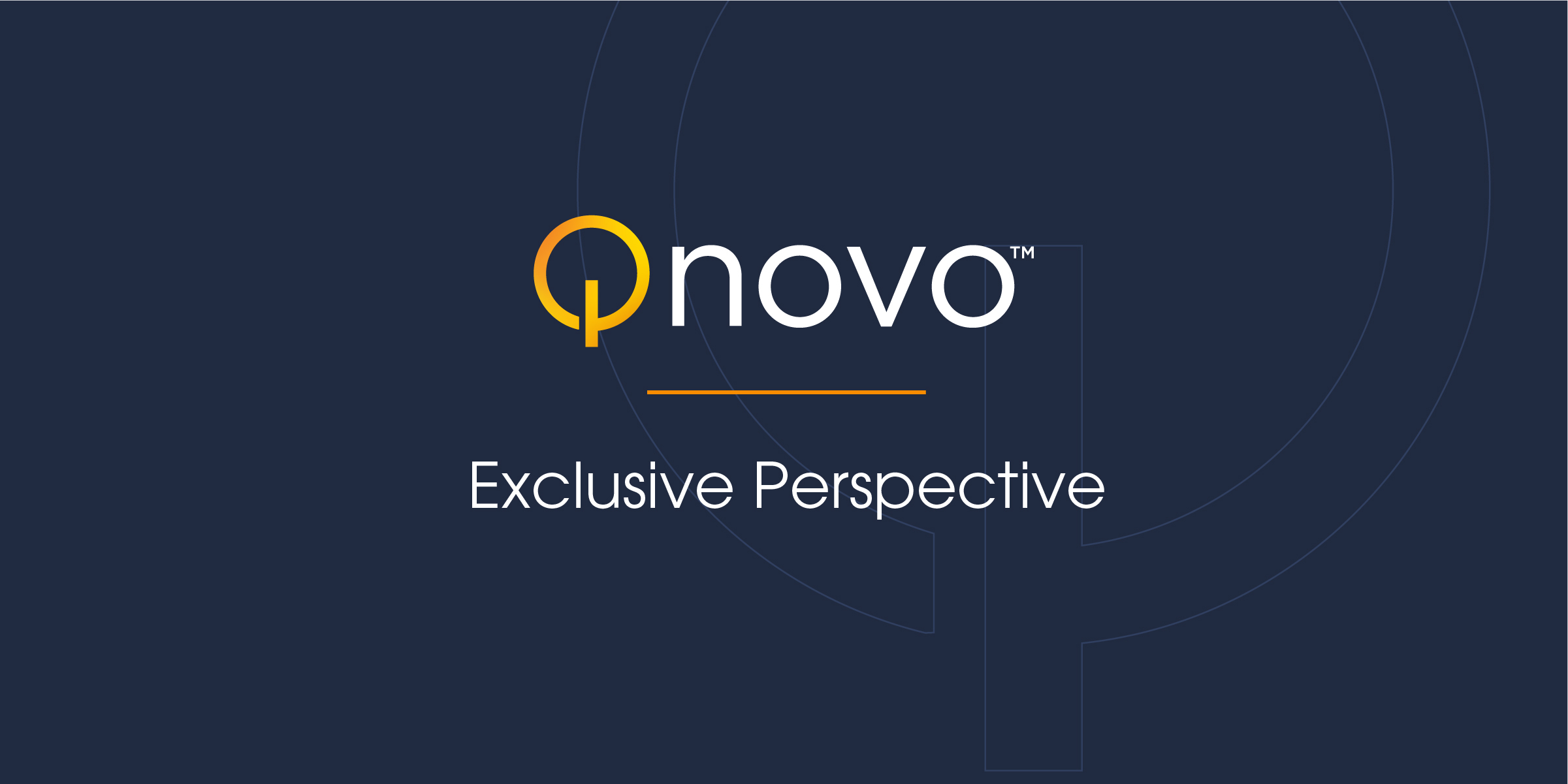
For the average reader, electrochemical impedance spectroscopy, often abbreviated as EIS, is more than a mouthful. Understanding its utility can be relegated to the category of unresolved mysteries. Today’s post will shed some light and a little intuitive thinking on this powerful method.
The reader’s first question might be “why are you talking about EIS in a battery blog?” The answer is simple. EIS is the foremost standard tool in laboratories around the world to measure electrochemical processes and reactions. Electrochemistry, one of the most extensive branches in chemistry, is the study of chemical reactions that have an inherent relationship to electricity, i.e. they can either generate electricity or can be influenced by electricity. Yes, you guessed right, batteries are a prime example of electrochemistry. Another practical example of electrochemistry put to good use: the gold plating on your necklace or bracelet.
What does the name EIS imply? Electrochemical impedance is scientific jargon that refers to the electrical resistance of the device under study, in this case, the lithium-ion battery. In its most elemental form, impedance is voltage divided by current. For electrical engineers, it represents components such as resistors or capacitors. For other scientists, it represents the resistance the device exhibits against the flow of electricity.
Spectroscopy is the branch of science that deals with how a property changes with frequency. Hence, EIS is the methodology and science that seek to understand how impedance measurements change with frequency, and more particularly, how these changes are intimately tied to the underlying chemical reactions.
Why frequency? Frequency adds a lot more information about the nature of the chemical process that is taking place. In science, frequency plays a very important role. Take for example the difference between blue and red light. They are both made of photons, but differ in frequency. Medical MRI imaging depends on the frequency of the oscillation of the hydrogen atoms in our bodies. Distinguishing between different broadcast stations on the radio dial operates on similar principles. In other words, we use frequency to uniquely identify chemical or physical processes.
With this long introduction, let’s dive a little deeper into EIS as related to a lithium-ion battery. If you were to measure the impedance of a standard electrical resistor component — the kind of components you may find inside your smartphone — you will find that you will measure exactly the same impedance value whether you apply a low voltage or a high voltage, or whether you measure at low frequency or high frequency. In other words, for this resistor component, the value is independent of voltage (also known as bias) and frequency. Resistors are consequently easy components to understand.
That is NOT the case for a battery. Change the voltage or frequency and you will get a different value. In other words, the battery can look like a resistor in some circumstances, or like a capacitor in others, or some complex combinations of both. When we change the voltage of the battery, it now operates at a different “state of charge,” in other words, it will have a different amount of electrical charge stored in it. As I described in this earlier post on fuel-gauges, the terminal voltage of the battery is a direct proxy of the amount of electrical charge stored in the battery, which is the state of charge (or the percentage of battery remaining).
In contrast, changing the frequency relates to different electrochemical processes that occur inside the battery. Such electrochemical processes could relate to the diffusion of the electrical charge (in this case, the lithium ions) from one electrode to the other. One can imagine that the ions have to travel a certain distance and insert themselves in the “Swiss-cheese” matrix of the material. So intuitively, this feels like a slow process, and it is. It takes several seconds to even minutes for the lithium ion to go through this diffusion process — meaning that diffusion of ions is characterized by a low-frequency signature. A distinctly different electrochemical process is how lithium ions and electrons interact right at the surface of the electrode. This interaction involves electrons and ions over very short distances. Intuitively, one can see that this can be a very fast reaction, usually on the order of microseconds. Hence its signature contains high frequency signals.

All of this goes to say that the impedance value at a particular frequency is a “unique signature” for the underlying electrochemical process of interest to our study. And that is what makes EIS such a powerful tool. To the trained scientist, he or she can read the EIS measurement as a map of the various electrochemical processes and reactions that are taking place inside the battery without cutting it open or damaging it. It also provides tremendous insight into what can also go wrong inside the battery. Not all electrochemical processes are desirable. For example, the underlying process that causes lithium metal plating is highly undesirable and can be readily measured using its unique EIS signature.
So how is the measurement made? In the laboratory, the oft-expensive and bulky instrument applies a small electrical current at a well defined frequency to the battery, then measures the voltage. Divide the voltage by the current and you now have the impedance at this frequency. For example, apply 1 mA of current at a frequency of 100 Hz, you might measure 0.5 mV. Hence the impedance is 0.5mV/1mA = 0.5 ohms at 100 Hz. This, of course, does not take into account the complex value of the impedance but it is a simple illustration of the concept. “Complex” numbers are mathematical tools to show values that have both real and imaginary components. Don’t worry if you don’t understand them fully —the key thing is that an impedance measurement has two values to represent it.
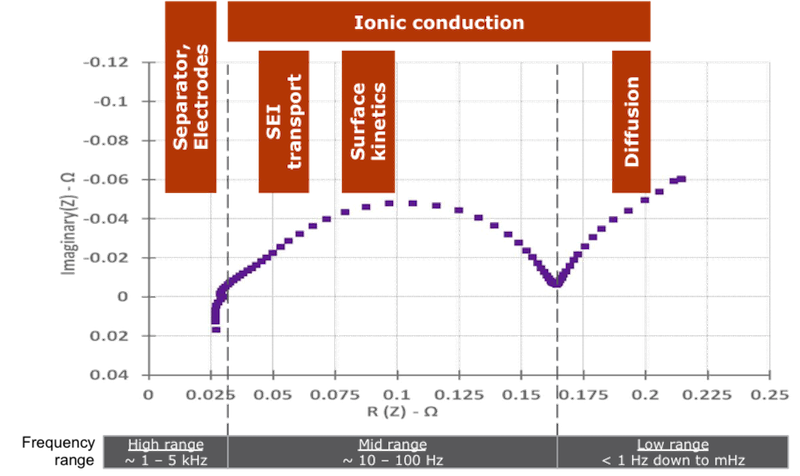
A full EIS chart shows by convention the imaginary component of the impedance (vertical axis) vs. its real value (horizontal axis). The far left of the chart shows the measurements made at high frequencies, in particular highlighting what happens in the metal conductors inside the battery as well as what occurs at the surfaces of the electrodes. As we follow the purple dots and move towards the right, the frequency of the signature gradually decreases highlighting now a different set of electrochemical processes, in particular what happens at the insulating interface between the electrode and the electrolyte (also known as SEI layer). Ultimately, to the far right of the chart, the frequency is low and is unique to the diffusion effects of the lithium ions.
An EIS tool is present in every electrochemistry laboratory around the world. Young graduates in this discipline spend countless hours operating this tool. It is not a small instrument…it fits on a desk, may weigh several pounds, and costs several thousands of dollars. Now imagine how the world would look like if an EIS tool can somehow fit inside each and every smartphone!