THE SCIENCE BEHIND WHY THE BATTERY VENDORS ARE HITTING THE WALL
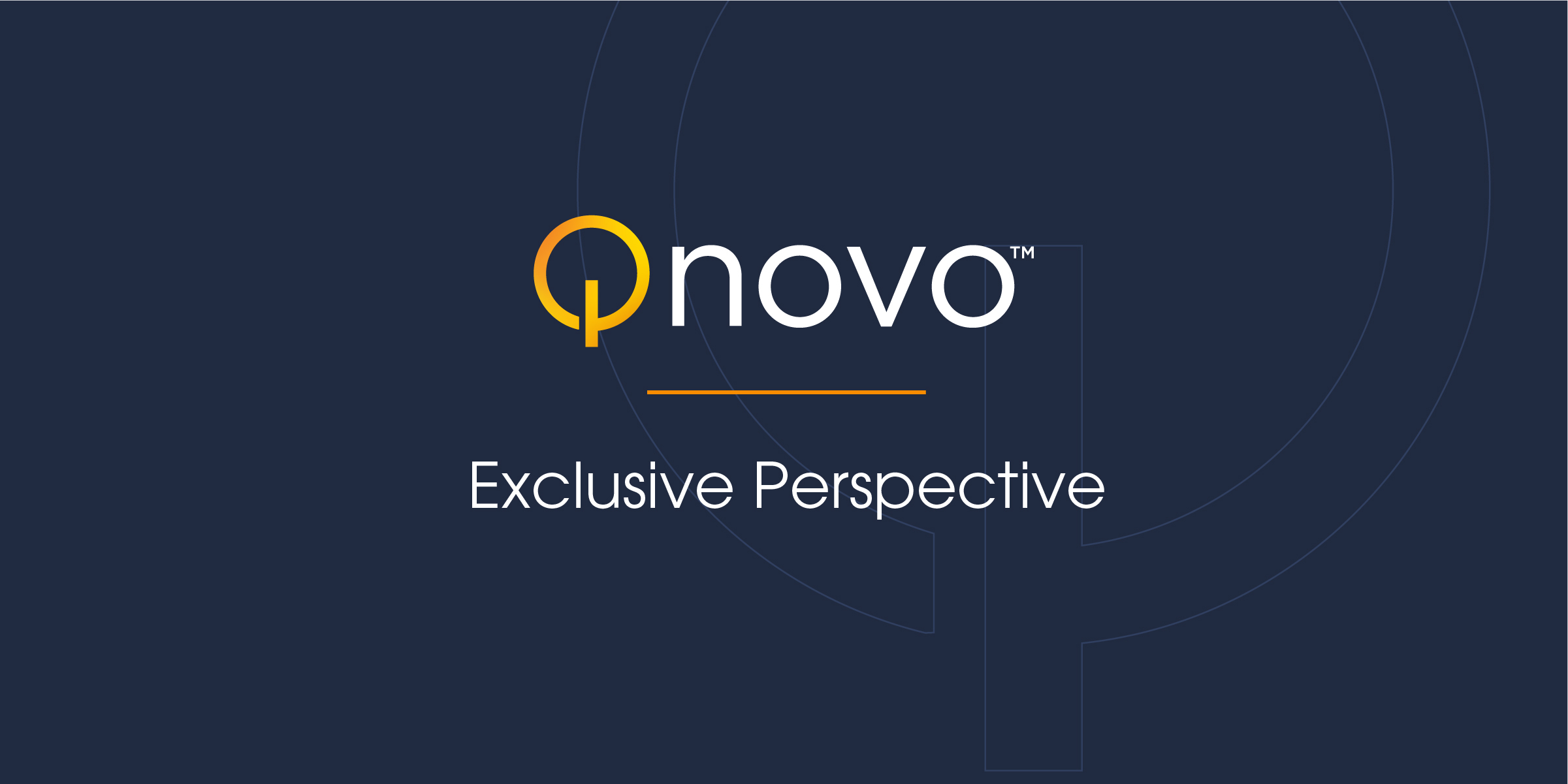
You have heard it before from me: battery performance is getting seriously challenged. The battery vendors are having a difficult time, and I am being kind, in meeting the expectations of the mobile device manufacturers. Specifically, it is getting extremely difficult for the battery vendors to simultaneously deliver high capacity, fast charging, and long cycle life. But why is that?
Let me digress briefly here and examine the average cruising speed of commercial airplanes over the past century. One will rapidly notice that airplanes have not gotten much faster in the last 50 years. They are more fuel efficient; they travel longer distances in greater comfort (well, if you pay for it); and they are far safer than they have ever been…but they are surely not faster. That’s because it gets very expensive to travel faster than the speed of sound (667 knots). The Concorde is a stark reminder of this economical limit. Chuck Yeager broke the physical limit of the sound barrier decades ago, but we can’t really change the economics around this limit.
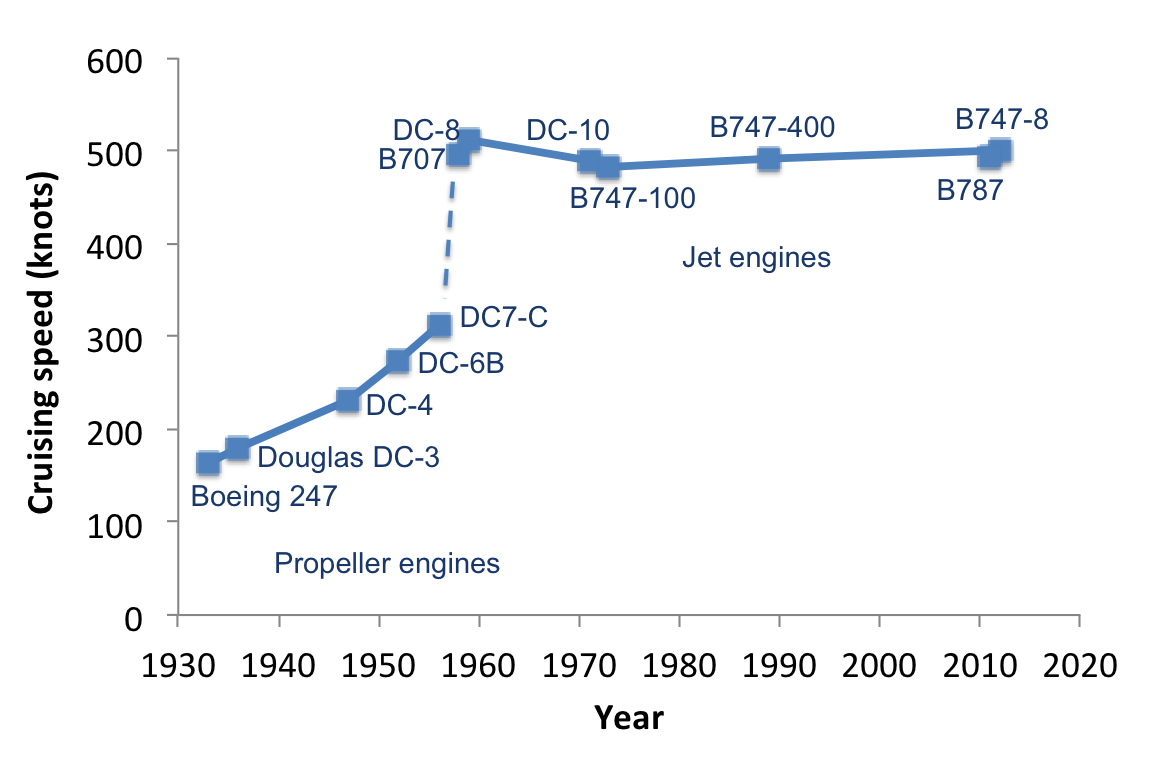
Lithium-ion batteries are rapidly approaching a similar limit for energy density. Short of a major breakthrough in a new material system, we are staring at a difficult barrier somewhere between 600 and 700 Wh/l. With that, I mean achieving large-scale manufacturing with affordable economics that match the requirements of the mobile device industry. A key limiting factor is now the carbon anode material. It is possible that new carbon-silicon composite anodes can change this equation, but for the foreseeable future, these new composites will continue to suffer from poor cycle life and high manufacturing costs. Until then, the economics of rising energy densities will be severely disadvantaged.
Is there a scientific origin for this limit? There are plenty of reasons, but it is best to illustrate one: the impact of battery voltage on energy density. In a very simplistic description, higher energy density comes from packing more lithium ions inside the battery, as well as raising the voltage at the terminals (if you recall, energy is the product of charge, or ions, and voltage).
The voltage at the terminals is the difference between two voltages; that of the cathode voltage in reference to a fictitious lithium contact, minus the voltage of the anode, also in reference to that same fictitious lithium contact. This is illustrated in the graph below.

On the vertical axis, I show the voltages of both the cathode vs. lithium (top) and the anode vs. lithium (bottom). On the far left of the chart, i.e., when x is approaching zero, the graphite is void of lithium ions and cobalt-oxide is totally full of lithium ions. This is when the battery is “empty.” On the far right, when x is approaching one, the opposite is true; the battery is “full.” I specifically refrain from saying x = 0 or x = 1. At these extremes, bad things happen. When x = 0, the physical structure of the cobalt-oxide alloy is greatly damaged. This limits the low end of the battery voltage to about 3.0 V. In other words, never discharge your lithium-ion battery below 3.0 V; the risk of irreversible damage is great. Most smartphones actually cut off near 3.3 V (this is really when your phone reads zero percent).
At the opposite end when x = 1, lithium ions combine to deposit (or plate) as a metal. The anode structure is also under immense mechanical stress. Additionally, when the cathode voltage rises past 4.2 V, the electrolyte begins to oxidize (and ultimately decompose). This effectively limits present-day lithium-ion batteries to a maximum voltage of 4.35 V with the understanding that the “bad stuff” begins to occur past 4.0 V, and becomes unsafe past 4.35 V.
To raise the energy density in the carbon/cobalt-oxide material system, one needs to raise the voltage and/or pack the electrodes as close as possible to each other. Well, raising the voltage past 4.35 V is getting very difficult. Finding electrolytes that can handle such voltages is no small feat. Additionally, the battery is now awfully close to the x = 1 point; in other words, the risk of lithium metal deposition at the anode is dangerously large at high energy densities. Life is getting tough, and there is very little room left for the battery vendors to maneuver.
These are just a few physical insights behind the challenges that the battery designers and manufacturers are facing. Finding solutions to these challenges via brute-force material development is not the answer. If you find yourself stuck with these limits, talk to us at Qnovo!